A few months ago, I started learning about a topic I've always been fascinated by: neuroscience. I took LessWrong's recommendation of the textbook Neuroscience: Exploring the Brain by Bear et al and started at the first page. I've always wanted to read a textbook front to back and I thought neuroscience would be a great topic to begin with.
I've now finished the first ten chapters, covering the fundamentals of neurons, neurotransmitters, and the sensory systems of sight, taste, and smell. In this post I'll share some of my high-level learnings and surprises so far about neuroscience as a field.
1. Membranes are all the rage.
Membranes are surprisingly important in neuroscience. A membrane is the separation between an entity and its environment. You may remember the phospholipid bilayer in high school biology class, which wraps around:
the cell
the nucleus inside the cell (containing DNA)
vesicles that contain material that needs to be transported within or between cells
organelles that perform functions within the cell (e.g. the mitochondria)
Membranes are ubiquitous: without them, an organism/cell/sub-cellular structure can't be anything distinct from its environment. Before reading this book though, my impression was that membranes were just there to establish this boundary, and all the fancy biology stuff happens inside cells.
But in neuroscience, a whole lot happens on the membrane itself. Possibly the most important role of the nervous system—the processing of information across a network of neurons—takes place across the membranes of neurons.
How does this work? Effectively, your neurons do a bunch of work to establish a small electrical imbalance between the inside and outside of the membrane:
We call this a resting potential. To transmit information, there's a brief reversal of the charge imbalance on the neuron's membrane—some positive particles move in—and this charge reversal ripples across the membrane like a wave. This is an action potential.
This is a simplified model; there's a whole lot of complexity in:
maintaining the resting potential
triggering an action potential under the right conditions
propagating the action potential across the membrane
transmitting information between neurons
...and in all of these functions, membranes play a vital role. So membranes are central in neuroscience.
2. All those pretty diagrams are fictions.
Part of the allure of reading an introductory textbook for me was all the colorful diagrams. I've always found them kind of beautiful.
One of the fascinating but disappointing takeaways has been seeing just how far from the truth these diagrams are. Take the diagram of a neuron:
This is as far as you might get without taking an actual neuroscience course. But in reality, here are some of our actual "pictures" of neurons:
Another example: this is a diagram of a synapse (the connection point between two neurons):
And here are some actual pictures of synapses:
The pictures are less appealing: fewer colors, more jagged curves, and less symmetry.
An especially striking example is with neurotransmitter receptors that lie on the membranes of neurons. These are proteins that act as "receivers" for messages from other neurons. Here's a diagram:
And here's a more detailed model of the receptor from wikipedia:
In all these cases, the more detail we consider, the messier things get.
Of course, this makes pedagogical sense—you should start with simpler diagrams to get the main ideas across, and slowly pile on more complexity as you go. But this was a good reminder that the cells in your brain are not as pretty, colorful, and smoothly delineated as you might imagine.
This layering of complexity brings me to my next learning:
3. It's always more complicated than you think.
Here's a common understanding I came into this textbook with: neurons are binary wires that transmit an electrical signal, functioning similarly to the architecture of my computer. In truth, neurons are much more complicated than bits. Consider:
A single neuron can have hundreds or thousands of synapses (connections with other cells)
A single synapse can have hundreds of postsynaptic densities, areas with clusters of receptor proteins
A single postsynaptic density can contain over a thousand proteins that serve multiple functions
The receptor protein is the atomic site of communication between cells. But a single receptor protein can trigger a complex, multifaceted response to a given neurotransmitter, bringing in many other proteins and chemical cascades.
So at each level of scale we find yet more complexity. Altogether, the neuron is a kind of brain in itself:
The signaling network within a single neuron resembles in some ways the neural networks of the brain itself. It receives a variety of inputs, in the form of transmitters bombarding it at different times and places. These inputs cause an increased drive through some signal pathways and a decreased drive through others, and the information is recombined to yield a particular output that is more than a simple summation of the inputs. (Chapter 6, Neurotransmitter Systems)
4. There's so much that we still don't know.
Before this book, I knew that we have so much to learn about high-level processing in the brain: perception, consciousness, emotion, thoughts. But it was exciting to learn that there are mysteries at all levels of granularity in the brain, including individual neurons. A few include:
Calcium and exocytosis: we don't fully understand how cells initiate the process of releasing neurotransmitters. We know the process involves calcium and the SNARE protein family but we don't know the details.
Glial cells: in addition to the 85 billion neurons in the brain, there are about as many glial cells. These cells fill the space between neurons, regulating the chemicals in extracellular space and insulating neuronal "wires". But some neuroscientists believe glia are the "sleeping giants" of neuroscience, potentially serving a more fundamental role than we realize. The astrocyte is an example of a glial cell whose function is an active area of research.
Sensory transduction: there is still much to learn about the exact processes by which inputs from the world (e.g. the contact of salt on our tongue) is converted into a neural signal. Example: while low salt concentrations trigger our salt receptors (as expected), high salt concentrations somehow trigger our bitter and sour taste receptors, and we don't know why. (This is why too much salt tastes bad.)
5. The same building blocks are used everywhere.
The brain, like other biological systems, evolved over many generations of natural selection and genetic variation. Evolution does not have much leeway in producing new systems entirely from scratch—it can only make incremental changes from its current state.
Learning about neuroscience is like reading multiple plot lines but with the same characters coming up again and again and playing slightly different roles.
Here's an example: glutamate is an amino acid.¹ Like other amino acids, it's used as a fundamental building block for making proteins in all of your cells. But within the nervous system, glutamate serves as the most common excitatory neurotransmitter. It's a neurotransmitter that excites the cells it contacts (as opposed to inhibiting them, like the neurotransmitter GABA).
But glutamate also serves a role in taste. Ever heard of MSG? It stands for monosodium glutamate, and the glutamate in it is what gives MSG the distinctive umami taste.
So in different contexts, glutamate serves the role of triggering our taste receptors, exciting neurons when transmitted between cells, or building proteins.
This brings me to an important point: many of the definitions in biology are functional rather than essential. A neurotransmitter is any molecule that acts as a signaling mechanism between neurons. Glutamate can act as a neurotransmitter, but it can also serve entirely different roles in other places.
6. Science takes time.
This was a stark contrast to my experience working in software startups. In technology (and especially software), it doesn't take that long to make a significant contribution and to get feedback about how well you're doing. You can build a prototype for a product and begin testing in a matter of weeks. Within a larger company, things might move a little slower, but it's almost impossible to spend a year on a project that goes absolutely nowhere.
Science is a totally different game. You could spend a decade working on a theory before learning it is wrong. It takes many years to fully flesh out a result, conduct the right experiments, get peer reviews, and let the dust settle until you learn whether your contributions will have any lasting impact at all.
It took decades to determine that most synaptic transmission between cells occurs via chemicals (neurotransmitters) rather than electrical charges. It took over 70 years to figure out whether neurons were in fact separate cells or one continuous reticulum (like the network of arteries and veins).
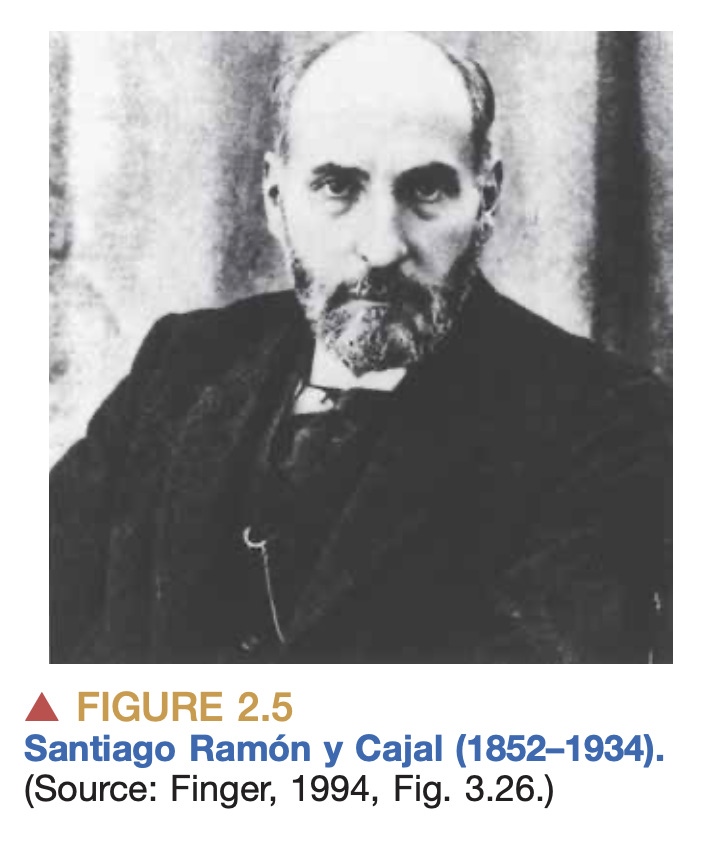
Conclusion
Those are some of the surprises and insights from my first foray into neuroscience: things you wouldn't know about the field until you dive deeper into it. I have another fifteen chapters to get through in this book, and the most exciting are yet to come: brain control of movement, the neural basis of behavior, motivation, language, and the learning and changing brain. In a future post, I might dig deeper into some of the technical learnings—how the eye works, the functions of different neurotransmitters, and so on. The journey continues!
If you found this post interesting, subscribe for more:
Notes
For the purposes of this discussion I'm treating glutamic acid and glutamate (which is its anion) as the same thing.